Table of Contents
Need of PRPG
Crop losses worldwide are largely caused by soil-borne diseases. Global crop productivity is adversely affected by diseases caused by plant pathogens, resulting in yield losses of 20-40 per cent in various cereal and legume crops each year.
Over the course of the 2016-17 agricultural season, 57,000 metric tonnes of synthetic pesticides were used in India to protect against plant pathogens and insect pests. However, biopesticides accounted for only 6340 metric tonnes.
Similar to weed herbicide resistance, many pesticide-resistant organisms have emerged due to the continued use of pesticides in modern agriculture and the presence of pesticide residues in vegetables, grains, and cereals also pose great dangers to human health.
the pesticides utilized to fight against plant illness affect the beneficial natural insect, soil fertility and soil microbiota adversely
Further, chemical pesticides and fertilizers used in an unregulated and indiscriminate manner cause pollution of the soil, water, and air, as well as a decline in soil fauna and microflora. To control soil-borne diseases around the world, large amounts of money are spent on synthetic pesticides. Due to the detrimental effects of synthetic chemicals on the environment, alternative approaches to plant disease control are being explored more and more.
the soil acidity due to these strong chemicals is also altered
*A Must Read : Types of soils
Anthropogenic activities are liable to cause ecological damage and deter soil health, ultimately depleting the nonrenewable assets. It is therefore essential to adopt various environment-friendly ways. In the present circumstance, sustainable agriculture is essential as it offers the capacity to meet not only our present needs but also ensure a healthy future, something that can’t be realized through the conventional harmful agrarian practices
In order to reduce the use of pesticides for agricultural crop production, beneficial rhizosphere microorganisms can be harnessed for sustainable agriculture solutions. In comparison with traditional chemical / synthesized pesticides, biopesticides offer several advantages, including 100% biodegradability and water solubility.
Plant-derived biochemicals and microorganisms are therefore a safer alternative for controlling plant disease in agriculture.
What is PGPR ?
Free-living plants growth-promoting rhizobacteria (PGPR) colonize the roots and soil surrounding plants, promoting their growth, development, and health.
A PGPR can also be classified as a biocontrol agent, a biofertilizer, or a biopesticide, depending on its activities/abilities.
Plant growth promoting rhizobacteria (PGPR) are critical players in agriculture
*A Must Read : Guide to biofertilizers
PGPR exerts its beneficial effects through the ability to control or prevent the spread of deleterious organisms that negatively impact plant health and growth.
It helps in crop well-being by fixing nitrogen, solubilising phosphate, reducing heavy metals, producing phytohormones (like auxin, gibberellins, cytokinins etc.), mineralising soil organic matter, decomposing crop residue, suppressing phytopathogens, etc.
Biocontrol of pathogens is done by PGPR in some of the following ways:
1. Some bacteria can colonize a plant niche faster and more effectively than disease-causing pathogens. This in turn results in low nutrient availability for the deleterious organisms as the beneficial bacteria competes for the nutrients.
2. Furthermore, some bacteria produce antibiotics. These antibodies are organic compounds that are lethal for disease-causing organisms in low concentrations.
3. Lastly, the bacteria induces a resistance mechanism in plants called Induced systemic resistance (IRS). This triggers the production of defence metabolites increasing the plant’s defensive ability.
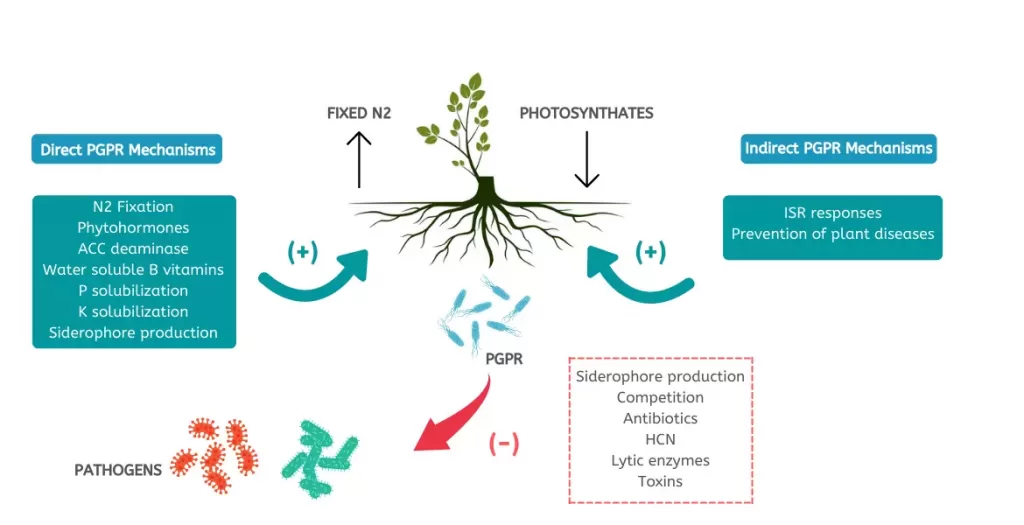
Advantages of PGPR
There are certain advantages to using PGPR as a biocontrol agent over chemical control compounds.
PGPRs are beneficial, naturally occurring microorganisms. They are also non-toxic and safe to use. Furthermore, from an ecological standpoint, they are sustainable (long term).
Furthermore, PGPRs possess a diverse range of modes of action, including antibiosis, siderophores, enzymes that degrade cell walls, bio-surfactants, and volatiles, as well as systemic resistance in plants.
PGPR against abiotic and biotic plant stresses
There are various types of stresses on the plant. These stresses can be broadly categorized into two types: Abiotic and biotic stresses.
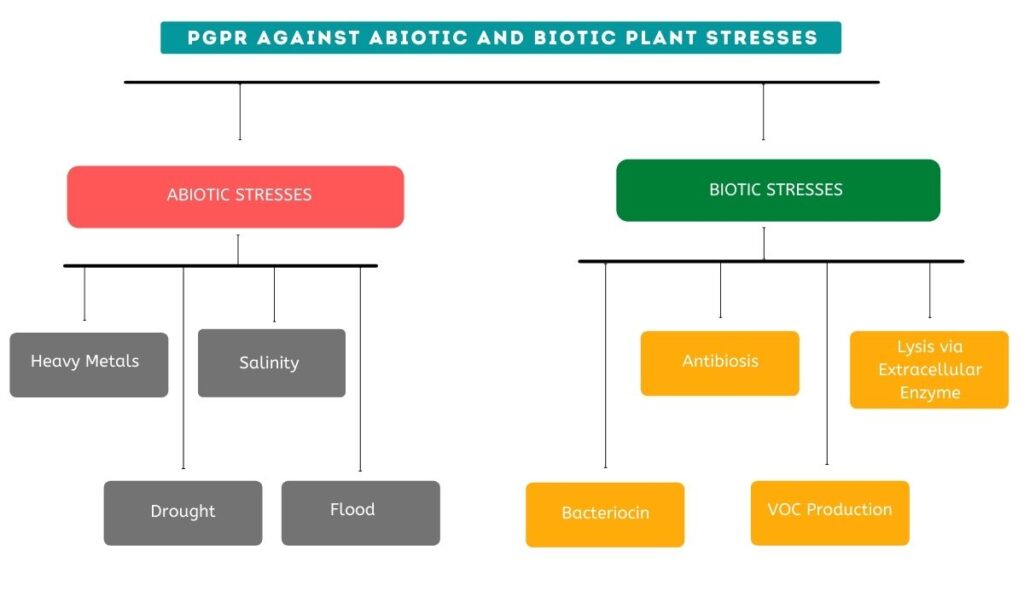
ABIOTIC STRESSES
Abiotic stress can be dictated by any unfavourable environmental condition that affects the diversity of microbes and also changes the physicochemical properties of the soil.
As part of abiotic stress, there are numerous conditions that have adverse impacts on the plant’s microbiome and surrounding ecologies, such as heavy metal toxicity, salinity, drought, and flooding.
- Heavy Metals
When noxious metals like mercury, asbestos, cadmium, and lead aggregate in soil, they cause plant stress and severely reduce crop productivity. In addition to negatively impacting soil pH and texture, metal aggregation directly affects a few biological processes in the soil, impairing crop growth.
The presence of metals in the rhizosphere delays plant growth by interfering with nutrient uptake.
Although PGPR increases plant growth and productivity, it also regulates soil metal contaminants through various mechanisms and improves soil properties.
The problem can be addressed by inoculating PGPR with metal resistance. Plants can be effectively protected from abiotic stress by PGPR by the induction of Induced Systemic Tolerance (IST).
A siderophores is a microbial metabolite that forms trace metal complexes. These are low molecular weight compounds that have good iron affinities. Microbes produce them when the iron is deficient in the soil. Metal stress is effectively resisted by siderophores produced by microbes.
Biosurfactants which are amphiphilic composites are mainly found on the surface of microorganisms. They improve trace metal tolerance and assist in removing soil metal.
Organic acids with low molecular weights like oxalic acid and citric acids are produced by PGPR. These organic acids reduce metal stress in farming. PGPR produces inorganic acids that could prevent metal stress through precipitation.
Extracellular polymeric substances (EPS) are heavy molecular weight homo- or hetero-polysaccharides microbial polymers. Rhizospheric bacteria release Extracellular polysaccharides such as lipopolysaccharides, polysaccharides, dissolvable peptides, and glycoprotein and create an anion restricting area that helps in the removal or detoxification of heavy metals through biosorption.
The following table shows the PGPR bioremediation of heavy metals in the soil:
PGPR | Plant | Metal(s) | Cultivation condition | Role of PGPR | References |
---|---|---|---|---|---|
Brevundimonas Diminuta, Alcaligenes faecalis | Scripus Mucronatus | Mercury | Green house | • Increased phytoremediation • Decrease in toxicity of soil | Mishra et al., 2016 |
Bacillus, Staphylococcus, Aerococcus | Prosopis juliflora, Lolium mltiforum | Chromium Cadmium, Copper, Lead and Zinc | Green house condition | • Improve the efficiency of Phytoremediation • Tolerate high conc. of • Chromium. | Wani and Khan, 2012 |
Rhizobium sp., Microbacterium sp. | Pisum sativum | Chromium (VI) | Glasshouse conditions | • Improve in concentration of nitrogen in the plants • Decreased Chromium toxicity | Mishra et al., 2016 |
Bacillus megaterium | Brassica napus | Lead | Under field conditions | • Decrease in Soil pollution • Total dry-matter yield of plants | Reichman, 2014 |
Bradyrhizobium japonicum CB1809 | Helianthus annuus and Triticuma estivum | Arsenic | Pot studies | • Excess of plant biomass • Growth in conditions of high arsenic concentration | Yavar et al., 2014 |
Mesorhizobium huakuii subsp. rengei B3 | Tomato Astragalus sinicus | Cadmium | Hydroponics | • Expression of PCSAt gene increased ability of cells to bind Cd2 | Sriprang et al., 2003 |
Bacillus subtilis SJ-101 | Brassica juncea | Nickel | Pot experiments in growth chamber | • Facilitated the accumulation of Nickel. | Zaidi et al., 2006 |
Azotobacter chroococcum HKN-5, Bacillus megaterium HKP-1, B. mucilaginosus HKK-1 | Brassica juncea | Lead, zinc | Pot experiments in greenhouse | • Stimulated plant growth • Protected plant from metal toxicity | Wu et al., 2006 |
- Salinity
It is detrimental for the agro-economy to have salinity conditions. As a result of long-term agrichemical use, salts are accumulating through soil over time, which leads to salinity issues.
Under salinity stress, reactive oxygen species (ROS) including O-2, O2, and H2O2 damage the cell, which is known as oxidative stress. A PGPR capable of producing both enzymatic and non-enzymatic components helps the plant to survive salt stress. By managing the H2O2 level, an enzymatic and nonenzymatic antioxidant system neutralizes such toxicity. ROS levels are routinely controlled by enzymes like catalase and ascorbate peroxidase and nonenzymatic components like ascorbate.
PGPR produce ACC (1-aminocyclopropane-1-carboxylate) deaminase, which protects plants from ethylene stress.
- Drought
A major factor hindering agricultural productivity worldwide is drought. Drought resistance refers to a plant’s ability to persist and endure during a drought.
By modifying the root architecture caused by bacteria, the total root area increases, resulting in improved nutrient and water absorption, which facilitates overall growth. The bacteria increases the number of rootlets with a lesser diameter with greater depth, hence increasing the total surface area of the root system.
With successful inoculation of PGPR strains, crop productivity may be enhanced by maintaining near-average shoot growth during drought stress. As it decreases the accessible leaf surface and evaporative loss of water is reduced.
Plant water status can be determined by measuring the relative water content (RWC). Plants treated with PGPR had better management of RWC than plants not treated with PGPR. PGPR can regulate the stomatal closure of a plant in dry areas and result in better RWC of a plant.
In plants, osmotic modification is a major adaptation strategy for dealing with drought-stress. In plants experiencing drought stress, proline is an important osmolyte. Plants with higher proline levels are more resilient to drought. Proline levels can be increased by PGPR inoculation.
Plant growth and development are facilitated by chemical growth regulators and phytohormones like cytokinins, abscisic acid, gibberellins, auxins, jasmonic acids (JAs) and ethylene. PGPR promotes the development of drought-stressed plants by regulating the phytohormones and growth regulators.
- Flood
During a flood, the exchange of gases in the root system reduces> this results in the accumulation of ethylene. Ethylene is responsible for regulating the flood-tolerant traits of plants. High ACC levels cause lower levels of oxygen in the root system during a flood.
The high concentration of ACC accumulated in the roots is reduced by ACC deaminase, which allows the ACC to diffuse out of the roots. This mechanism reduces the levels of ethylene during and after flooding.
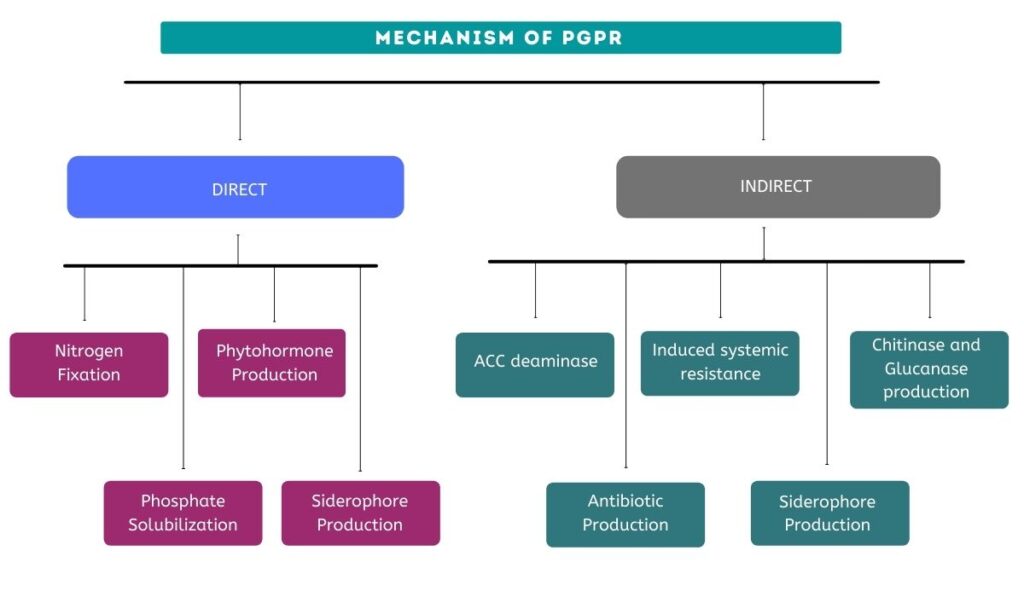
BIOTIC STRESSES
In plants, biotic stress is caused by living organisms, such as bacteria, viruses, fungi, insects, and nematodes. These organisms interfere with host nutrients, resulting in plant death. Biotic stress contributes to both pre- and post-harvest losses.
Although few microbes participate in the biological control of pathogens, yet PGPR is known to create protection from many diseases following various mechanisms including bacteriocin, antibiosis, volatile organic compound (VOC) production, and lysis through the extracellular enzyme
- Bacteriocin
Bacterial toxins against bacteria aka Bacteriocins are peptide secretions with limited antimicrobial activity. Bacteriocins are produced by both Gram-negative (for example, colicin) and Gram-positive bacteria (for example, nisin). Under laboratory conditions, bacteriocins have been shown to be beneficial in fighting tomato bacterial spot disease.
- Antibiosis
Because of their antimicrobial, insecticidal, antiviral, phytotoxic, cytotoxic, and antihelminthic properties, PGPR antibiotics are more powerful than others. A wide range of antifungal substances are produced by Pseudomonas, including 2,4 diacetylphloroglucinol (2,4-DAPG), butyrolactones, rhamnolipids, N-butylbenzene sulfonamide.
- VOC Production
There are numerous Volatile Organic Compounds (VOC) secreted by the PGPR that are biocontrol specialists for certain nematodes and microorganisms. VOCs include benzene, cyclohexane, tetradecane, and 2-(benzyloxy)-1-ethanamine. HCN is one of the VOCs (delivered by rhizospheric microbes) that is capable of controlling some phytopathogens.
- Lysis via Extracellular Enzyme
PGPR produces lytic compounds that enable plants to fight infection-causing microbes. Rhizobacteria produce extracellular enzymes (chitinase and β-1,3- glucanase) that are linked with the lysis of the cell wall. Chitinase and β-1,3-glucanase are strong antifungal compounds on the fungal cells having their walls made of chitin and β−1,4-N-acetyl-glucosamine.
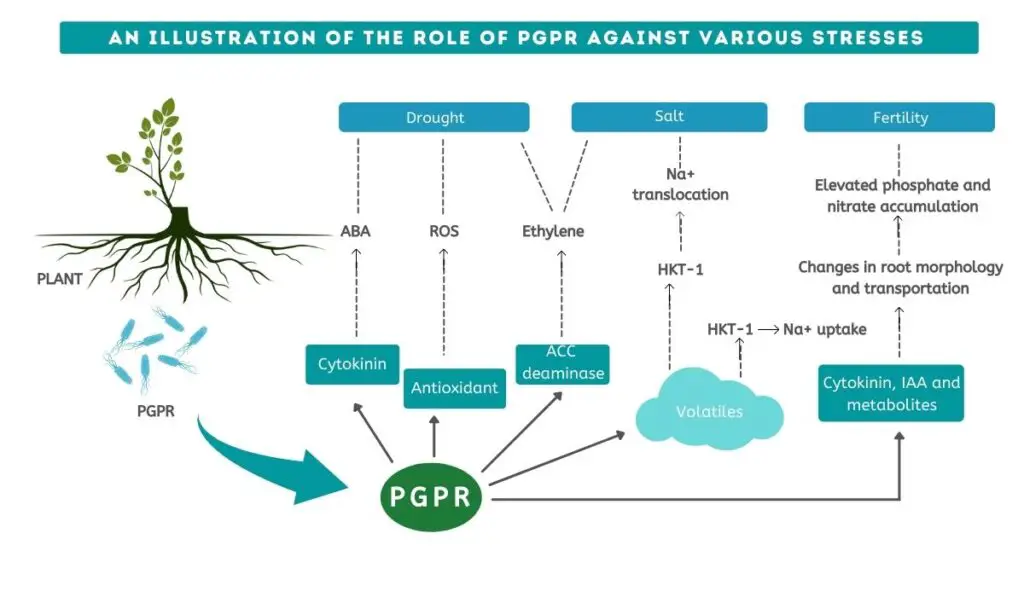
Symbiotic relation of PGPR and plants
A metabolic interaction between plants and microbes is proposed to involve amino acids, sugars, organic acids, and other carbon sources provided by plants.
Metabolic associations between plants and rhizomicrobes can be explored in this niche.
Biological metabolites from rhizospheric microorganisms are crucial to ecological success. According to their substrate uptake patterns, rhizomicrobes in this habitat play an important ecological role.
Many rhizobacteria strains function in a way that excretes a novel metabolite that is not found in the native root system. Hence complimenting the root system.
By adding ample amounts of sugar, soil microbes rapidly proliferate, giving the impression that carbon in the soil is in a limited capacity in the soil. Thus, it is attributed that plants contain ample levels of carbon that diffuse to the outside through multiple metabolic pathways.
Although plants fix carbon primarily through respiration, rhizospheric deposition also releases carbon.
Rhizobacteria produce metabolites for plants, but rhizodeposits in plants produce a range of metabolites which offer huge opportunities both to attract and inhibit specific bacterial strains.
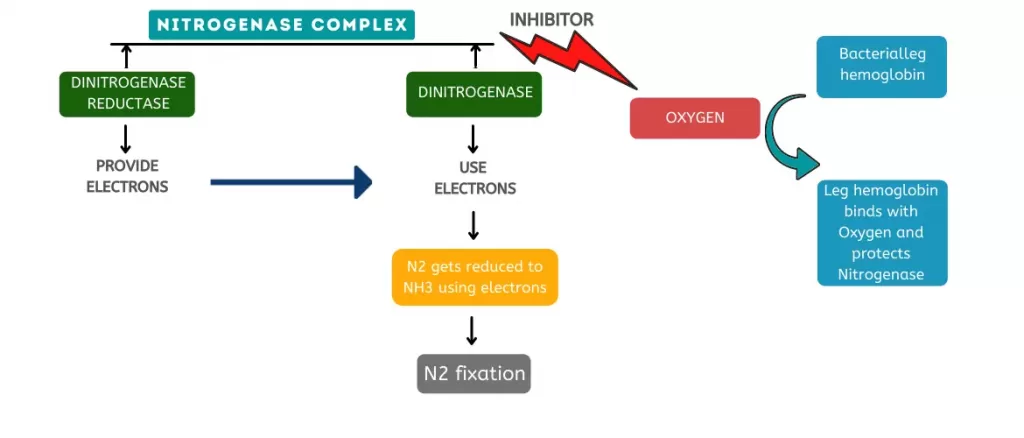
Rhizomicrobes supply nitrogen, phosphorus, and iron to plants in utilizable forms that are essential for plant growth.
Rhizomicrobes produce phytohormones such as ACC deaminase, cytokinin, and indole-3-acetic acid that are essential for plant growth and development.
Criteria of selection of PGPR
For the development of a successful PGPR formulation, therhizobacterial species should possess the following characteristics
- Enhance the growth of plants
- Multiplication on a mass scale should be possible
- Must possess high rhizospheric competence
- Demonstrate high competitive saprophytic ability
- Demonstrate a broader range of activities
- Ecological compatibility with other rhizobacteria
- Resilient to abiotic stress conditions (thermal, radiation, desiccation and oxidizing agents)
- Should be environmentally friendly
PGPR as biofertilizer
Biofertilisers are live formulations of beneficial microbes that assist in making nutrients available to plants. Through its biological activity, it improves soil health and thus the soil microflora.
Is PGPR as biofertilizer?
Yes. PGPR is a bio fertilizer.
PGPM are the main component of this biofertilizer. The PGPM can be classified into three major groups, namely, arbuscular mycorrhizal organisms (AMF), plant development advancing rhizobacteria (PGPR), and nitrogen fixing rhizobia.
PGPR has been utilized worldwide as a biofertilizer, increasing yields and soil quality. With PGPR likely to be committed, it could lead to sustainable agribusiness.
These biofertilizers are available in solid as well as liquid forms, with liquid formulations being found to be more effective. Root inoculation, seed inoculation, and soil inoculation are the three main types of liquid formulations.
Upon applying Burkholderia phytofirmans biofertiliser to Ryegrass root, seed, and soil, soil inoculation method was most efficient in improving the production of plant biomass, phytoremediation and hydrocarbon degradation
Constraints of PGPR
- PGPR have a natural variation property that limits their use. Under field conditions (as opposed to controlled laboratory conditions), it is difficult to predict how an organism will behave.
- In addition, PGPRs are living microorganisms, so having the ability to propagate them artificially in the field is another challenge.
- Mass production in an optimized manner is also a challenge. Furthermore, maintaining their durability and efficiency of biological activity until field application is also tricky.
- PGPR bacteria are not able to live in soil for very long, and over time cultivators will need to reinoculate to maintain their population in the field.
Examples of PGPR
What is PGPR example?
Following are some of the examples of PGPR and their roles in rhizosphere:
Representative species | Role | Mechanism(s) involved | Participating plant(s) | Reference(s) |
---|---|---|---|---|
Agrobacterium radiobacter | Improves bioprotection | Antibiotics | - | Mohanram and Kumar, 2019 |
Azotobacter chroococcum | Assists in biostimulation | Production of gibberellin | Cereals | Zhang et al., 2019 |
Aids in bioprotection | Siderophore | - | ||
Azospirillum brasilense | Biofertilisation | Phosphate solubilisation | Maize (Zea mays), Wheat (Triticum aestivum L.) and Rice (Oryza sativa) | Lucy et al., 2004 |
Bacillus cereus | Boosts bioprotection | Lipopeptides | Bean (Phaseolus vulgaris) | Ongena and Jacques, 2008; Vaikundamoorthy et al., 2018; Hashami et al., 2019 |
Induced and acquired systemic resistance | Tomato (S. lycopersicum) Soybean (Glycine Max L.) | |||
Bioremediation | Production of amylase Remediation of industrial waste | - - | ||
Bacillus subtilis | Biofertilisation | Ammonia synthesis | Maize (Zea mays) | Ouhaibi-Ben Abdeljalil et al., 2016; Ait-Kaki et al., 2014; Tahir et al., 2017 |
Aids in biostimulation | Through IAA and Cytokinin production | Chickpea (Cicer arietinum) Tomato (S. lycopersicum L.) | ||
Bioprotection | Lipopeptides | - | ||
Catalase production | Cucumber (Cucumis sativus) | |||
Bioremediation | Degrading xenobiotics and allelochemicals | - | ||
Enterobacter oryzae | Biofertilisation | Nitrogen fixation | Mangart and Jam (Acacia acuminate) | Dinnage et al., 2019 |
Frankia casuarinae, F. inefficax, F. irregularis, and F. saprophytica | Biostimulation | Cytokinin production | - | Nouioui et al., 2019 |
Klebsiella pneumonia | Aids biofertilisation | Nitrogen fixation | Maize (Zea mays) | Kuan et al., 2016; Sharma et al., 2019 |
Bioprotection | Acquired and induced systemic resistance | Peanut (Arachis hypogaea) | ||
Mesorhizobium loti | Biofertilisation | Nitrogen fixation | Lotus (Arabidopsis thaliana) | Kaneko et al., 2000 |
Methylobacterium exotorquens | Assists biostimulation | Cytokinin output | Arabidosis, barley, maize and soyabean | Kaneko et al., 2000 |
Paenibacillus xylanexedens | Facilitates bioprotection | Chitinase production | Wheat (Triticum aestivum L.) | Verma et al., 2016 |
Pseudomonas aeruginosa | Assists in biofertilisation | Phosphate solubilisation | Maize (Zea mays) | Hameeda et al., 2008; Ahemad and Khan, 2012; Paramanandham et al., 2017; Cheng et al., 2019; Lawrance et al., 2019 |
Aids in bioprotection | Ammonia production | Field mustard (Brassica campestris L.) | ||
Hydrogen cyanide production | Elephant grass (Pennisetum purpureum) | |||
Assists in Bioremediation | Cellulase production | Rice (O. sativa), Pea (P. sativa) | ||
Heavy metals uptake | Amaranthus, Tomato (Solanum lycopersicum L.) | |||
Rhizobium leguminosarum | Biostimulation | Gibberellin production | Rice (O. sativa L.) | Yanni et al., 2001 |
Serratia marcescens | Bioprotection | Producing siderophore, chitinase and protease | Field pumpkin (Poa pratensis) | Selvakumar et al., 2008; Rathore and Gupta, 2015 |
Staphylococcus saprophyticus | Biostimulation | Manufacturing of IAA | Ornamental species | Manzoor et al., 2019 |
Stenotrophomonas rhizophila | Bioprotection | Amylase synthesis | Maize (Zea mays) and Canola (Brassica napus) | Ghavami et al., 2017 |